Post by Andrei Tchentchik on Jun 24, 2019 16:16:34 GMT 2
(.#217).- Hypothesis of the rare earth.
Hypothesis of the rare earth.

Are the planets able to shelter life like the Earth rare?
In planetary astronomy and astrobiology, the rare earth hypothesis holds that the emergence of a complex multicellular life (metazoa) on Earth has required an unlikely combination of astrophysical and geological events and circumstances. The term rare earth comes from Rare Earth: Why Complex Life Is Uncommon in the Universe (2000), a book written by geologist and paleontologist Peter Ward, and astronomer and astrobiologist Donald E. Brownlee. Their book is the source of the essence of this article.
The rare earth hypothesis is opposed to the principle of mediocrity (also called Copernican principle) supported by Carl Sagan and Frank Drake, among others1. The principle of mediocrity concludes that the Earth is a typical rocky planet, in a typical planetary system, located in a region without any exceptional character of a current barred spiral galaxy. It is therefore likely that the universe teems with complex life. Ward and Brownlee argue that planets, planetary systems, and galactic regions that support a complex life, such as the Earth, the Solar System, and our Milky Way region, are probably very rare.
The rare earth hypothesis, concluding that complex life is not common, is a possible solution to the Fermi paradox: "If extraterrestrials are common, why do not we see them? "
Galactic habitable areas
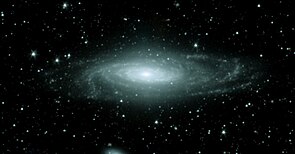
In a zone lacking metals or near the center of the galaxy and exposed to significant radiation, a planet is not able to shelter life. The galaxy photographed is NGC 7331, often identified as the twin of the Milky Way.
Rare Earth suggests that much of the known universe, including large parts of our galaxy, can not harbor complex life; Ward and Brownlee refer to these areas as "dead zones". Parts of galaxies where complex life is possible constitute the habitable galactic zone. This area is primarily a function of the distance to the galactic center. With increasing distance:
1. The metallicity of stars declines, and metals (term which, in astronomy, means elements heavier than hydrogen and helium) are necessary for the formation of telluric planets.
2. X and gamma radiation from black holes in the center of the galaxy, and near neutron stars, are losing intensity. A radiation of this nature is considered dangerous for the complex life, so that the rare earth hypothesis predicts that the primitive universe, and also now, the regions of the galaxy where the stellar density is high and the supernovae are unsuited to the development of complex life.
3. The probability of gravitational disturbances to planets (and planetesimals) by nearby stars decreases with the decreasing density of stars. So the more distant a planet is from the center of the galaxy, the less likely it is to hit a big car. A sufficiently large impact can lead to the extinction of all complex life on a planet.
Proposition (1) above governs the outer reaches of a galaxy; propositions (2) and (3) govern the inner regions of the galaxy, the globular clusters and the spiral arms of the spiral galaxies. These arms are not physical objects, but regions of a galaxy characterized by a high rate of star formation, moving at a speed of about 240 km / s (for the solar system), they describe a movement wave. From the center of a galaxy to its periphery, the capacity to shelter life increases and then falls. So that the habitable galactic zone can affect the shape of a ring, sandwiched between its center and its periphery, both uninhabitable.
Not only can a planetary system enjoy a location favorable to the appearance of complex life, but it must also maintain this location for a period long enough for the complex life to evolve. Thus, a central star with a galactic orbit that evolves away from galactic regions with high levels of radiation such as galactic centers and spiral arms seems potentially a very favorable support. If the galactic orbit of the central star is eccentric, elliptical or hyperbolic, it will cross a few spiral arms, but if the orbit is a nearly perfect circle, and the orbital velocity equals the speed of "rotation" of the spiral arms, the star will eventually sink into a spiral arm region, first gradually, then fully. Therefore, the supporters of the "Rare Earth" conclude that a life-supporting star must have a nearly circular galactic orbit around the center of its galaxy. The required synchronization between the orbital velocity of a central zone star and the velocity of the spiral arms wave can occur only in rather narrow bands of distances to the galactic center. These regions are referred to as the "habitable galactic zone". Lineweaver et al. calculate that the habitable galactic zone is a ring of 7 to 9 kiloparsec in diameter, which comprises not more than 10% of the stars of the Milky Way. Based on conservative estimates of the total number of stars in the galaxy, this could be in the range of 20 to 40 billion stars. Gonzalez et al. reduce this figure by half, they estimate that at most 5% of the stars of the Galaxy are in the galactic zone habitable.
Indeed, the Sun's orbit around the center of the Milky Way is a nearly perfect circle, with a period of revolution of 225 million years, closely matching the rotation period of the galaxy. Karen Masters has calculated that the Sun's orbit crosses a major spiral arm every 100 million years. On the contrary, the rare earth hypothesis predicts that the sun, since its formation, should not have passed through any spiral arms. However, some researchers have suggested that several mass extinctions actually correspond to previous spiral arm crossings.
A central star with the adequate character
The example of the Earth suggests that complex life requires the presence of water in the liquid state and its planet must therefore be at the appropriate distance from its star. This is the heart of the concept of habitable zone or principle of Goldilocks (in). The habitable zone forms a ring around the central star. If the orbit of a planet is too close to or too far from its sun, the temperature of its surface is incompatible with water in liquid form (although water below the surface, as has been suggested for satellites Europe and Enceladus, as well as for the dwarf planet Ceres can exist in different places). Kasting et al. (1993) estimate that the habitable zone of the Sun extends between 0.95 and 1.15 astronomical units.
The living area varies with the type and age of the central star. The habitable zone of a star in the main series drifts with time until the star becomes a white dwarf, when the habitable zone disappears. The habitable zone is closely linked to the greenhouse effect caused by atmospheric carbon dioxide (CO2) or other greenhouse gases, mainly water vapor in the case of the Earth. The collective effect of these gases increases the average temperature on Earth by approximately 33 ° C compared to what it would otherwise be.
It is assumed that a star must have rocky planets within its habitable zone. While the habitable zone of hot stars such as Alpha Canis Majoris (Sirius) or Alpha Lyrae (Vega) is wide, there are two problems :
1. Since it was thought (at the time of writing "Rare Earth") that the rock planets were forming closer to their central stars, the candidate planets would then form too close to the star to find themselves in the habitable zone. This does not solve life on a gas giant moon. Hot stars also emit a lot more ultraviolet radiation that will ionize any planetary atmosphere.
2. The hot stars mentioned above have a short life span, becoming red giants in less than a billion years. This time is not enough for an advanced life to evolve. These considerations remove stars of types F6 to O (see spectral type as the focus of evolution of an evolved metazoan life.
On the other hand, small stars (red dwarfs) have small radius living areas. This proximity means that one side of the planet is constantly facing the star, and the other remains permanently in the dark, a phenomenon of "lock" due to the effects of tidal forces. This "tidal locking" of a hemisphere from a planet to its primary body will make the diurnal face of the planet constantly hot, while the night face will remain extremely cold, even cold enough so that most atmospheric gases can freeze! Planets within a liveable area with a small radius are also subject to increased risks from solar flares (see Aurelia), which will tend to ionize the atmosphere and be unfavorable to the complex life of the planet. multiple ways. Proponents of "Rare Earth" hold that this eliminates the possibility of living in such systems, while exobiologists have suggested that livability may exist in the correct circumstances. This is a central blocking point for the theory, since these late K-type stars and M constitute about 82% of all hydrogen-consuming stars.
Proponents of "Rare Earth" argue that the type of central stars that are "just right" ranges from F7 to K1. Such stars are not common: G-type stars like the Sun (between the warmer F and the colder K) only comprise 9% of the hydrogen-consuming stars in the Milky Way.

Globular clusters probably do not house life.
Older stars such as red giants and white dwarfs probably do not contain life either. Red giants are common in globular clusters and elliptical galaxies. White dwarfs are essentially dying stars that have already gone through their red giant phase. The diameter of a red giant has increased substantially since his youth. If a planet has been in the habitable zone of a star during its youth and middle age, it will be burnt when the star becomes a red giant (although theoretically the planets farther away might have become habitable) .
The energy expenditure of a star throughout its life changes only very gradually: variable stars such as, for example, the Cepheid variables are extremely unlikely hosts of life. If the energy expenditure of the central star decreases suddenly, even for a relatively short time, the planet's water can freeze. On the other hand, if the energy expenditure of the central star increases significantly, the oceans can evaporate, which produces a greenhouse effect: this phenomenon can eliminate any reformation of the oceans.
There is no known way to achieve life without complex chemistry, and such chemistry requires metals (which means heavier elements than hydrogen, helium and lithium). This suggests that one of the conditions for life is a solar system rich in metals. The only known mechanism for the creation and dispersion of metals is a supernova explosion. The presence of metals in the stars is revealed by their absorption spectrum, and stellar spectra studies reveal that many stars, and perhaps most of them, are low in metals. Low metallicity characterizes the primordial universe, globular clusters and other stars formed during the youth of the universe, stars in most other galaxies as large spiral galaxies, and stars in the outer regions of all galaxies . Thus, metal-rich central stars capable of harboring a complex life are estimated to be very common in the quiet suburbs of larger spiral galaxies, hospital regions with complex life, for another reason, specifically the absence of high radiation.
A planetary system
A cloud of gas capable of giving birth to a star can also give birth to a gaseous planet with low metallicity (Jovian) like Jupiter and Saturn. But the Jovian planets do not have a firm surface, considered necessary for the complex life (their satellites, however, can have hard surfaces). According to Ward and Brownlee's argument, a planetary system capable of harboring complex life must be structured more or less like the Solar System, with the small rock planets inside and the Jovian planets outside. However, recent research questions this line of argument.
Uncertainty about Jupiter

Jupiter is the fifth planet from the Sun and the largest planet inside the Solar System.
At the time of Ward and Brownlee's book, gaseous giants were seen as helping to sustain life, retaining distant asteroids from the planets that harbor life. So it was thought that a gas giant was protecting inner rocky planets against asteroid bombardment. However, recent computer simulations on the subject suggest that the situation is more complex: it appears that Jupiter causes three times more impacts than it avoids, while the replacement of Jupiter by a body the size of Saturn , would double the rate of bombing compared to that obtained with Jupiter.
Orbital disturbance
A gas giant must not be too close to a body on which life develops, unless that body is one of its moons. The position too close to a gas giant could disrupt the orbit of a potentially life-giving planet, either directly or by drifting into the habitable zone.
Newtonian dynamics can produce chaotic planetary orbits, especially in a system with large planets with high orbital eccentricity.
The need for a stable orbit is imposed on extra-solar planetary systems composed of large planets with orbits near the star, known as hot Jupiters. Hot Jupiter are thought to have formed much larger distances from their parent-star than they currently are, and have migrated inward to their present position. In this process, they will have catastrophically disrupted the orbit of all planets in the habitable zone.
Global dimension
A planet too small is not able to retain enough atmosphere. Changes in surface temperature would be much larger, and the average temperature would decrease. The formation of substantial oceans and their long-term maintenance would be compromised. The surface of a small planet will also tend to be very rugged, with high mountains and deep canyons. His heart will cool too quickly, and plate tectonics either will not last as long as on a larger planet, or will not occur at all.
According to astronomer Michaël Meyer of the University of Arizona, small planets like the Earth may be common: "Our observations suggest that between 20% and 60% of Sun-like stars show signs of rocky planets, little different from the processes we think they led to the appearance of planet Earth. It's very exciting. ". The team led by Meyer has highlighted the presence of cosmic dust near solar-type stars, and sees this as a by-product of rocky planet formation.
A big moon
The Moon is unusual because the other rocky planets of the Solar System either do not have a natural satellite (Mercury and Venus), or have tiny, presumably captured asteroids (Mars).
The theory of giant impact formulates the hypothesis that the Moon results from the impact of the planet Theia (the size of Mars) with the Earth during its youth. This giant collision also gave the Earth its inclination of the axis and its speed of rotation. A fast rotation reduces the temperature change over the course of a day and makes photosynthesis viable. The rare earth hypothesis continues with the argument that the inclination of the axis should not be too great in relation to the orbital plane. A planet with too much inclination would withstand extreme seasonal climatic variations, unfavorable to complex life. A planet with little or no inclination would lack a stimulus to evolution provided by climatic variations. In this perspective, the tilt of the Earth is "just right". The gravity of big satellites also stabilizes the tilt of the planet; without this influence, the inclination would become chaotic, possibly rendering impossible the complex terrestrial forms of life.
If the Earth did not have a moon, the ocean tides resulting solely from the gravitational influence of the Sun would be very modest. A large satellite engenders the existence of residual pools, which may be essential to the formation of complex life.
A large satellite also increases the likelihood of plate tectonics by the effect of tidal forces on the planet's crust. The impact of the Moon may also have had an initiating role of plate tectonics, without which the continental crust would cover the entire planet, leaving no room for the oceanic crust [ref. necessary]. It is possible that large-scale convection of the mantle, necessary prior to the appearance of plate tectonics, could not have emerged in the absence of inhomogeneity of the crust. However, there are strong signs that plate tectonics existed on Mars in the past, without such a mechanism initiating it.
If a giant impact is the only way for an inner rocky planet to acquire a big satellite, any planet in the circumstellar habitable zone will need to form as a double planet, so that there is a sufficiently massive object to generate an impact to generate a large satellite in a timely manner. An "impacting" object of this nature is not necessarily improbable. Recent work by Edward Belbruno and Princeton University's John Richard Gott suggests that an adequate "impacting" body could be formed at Lagrange points on a planet.
Plate tectonics
A planet can only have tectonic plates if its chemical composition allows it. The only known long-term source providing the necessary heat is the radioactivity that occurs in the depths of the planet. The continents must also consist of granitic rocks that float on denser basaltic rocks. Taylor points out that subduction zones (an essential part of tectonic plates) require the lubricating action of large amounts of water; on Earth such areas exist only in the ocean floor.
Episode of the inertial upheaval
There is enough evidence that the rate of continental drift during the Cambrian explosion was abnormally high. In fact, the continents moved from the Arctic to the equator and vice versa in 15 million years or less. Kirschvink et al. have proposed the following controversial explanation: a 90 ° change in the axis of rotation of the Earth, caused by an imbalance of the continental masses with respect to the axis. The result was an enormous change in climate, ocean currents, and so on, occurring in a very short time and affecting the entire Earth. They named their explanation as "the episode of inertial upheaval".
This scenario has not yet been endorsed by the scientific community, but if such an event really happened, then, it was very implausible, and if it was a necessity for the evolution of an animal life more complex than sea sponges or coral reefs, then we still get a new reason for life (complex?) to be rare in the universe.
Rare Earth equation
The following is adapted from Cramer. The rare earth equation is the riposte
from Ward and Brownlee to Drake's. It allows the calculation of N, the number of Earth-like planets in the Milky Way with complex life forms.
The rare Earth equation, unlike Drake's, does not factor in the probability that complex life evolves into intelligent life that discovers technology (remember that Ward and Brownlee are not evolutionary biologists). Barrow and Tipler observe the consensus among these biologists that the path of evolution from primitive Cambrian chordates (eg Pikaia) to homo sapiens was a highly improbable event. For example, the large brains of humans are the mark of adaptive disadvantage, since they require an expensive metabolism, a long period of gestation, and a duration of youth of more than 25% of the average total duration of the life. Other improbable traits in humans include :
• Being the only vertebrate non-avian terrestrial bipedarian of great extent. Combined with an unusual eye-hand coordination, this allows skillful manipulation of the physical environment with the hands;
• A vocal apparatus much more expressive than that of any other mammal, allowing speech. Speech allows humans to interact cooperatively, share knowledge, and acquire a culture;
• The ability to formulate abstractions to a degree that allows the invention of mathematics, and the discovery of science and technology. It must be kept in mind that humans have recently acquired all that makes up the sophistication of their scientific and technological knowledge.
Supporters
Books supporting the rare earth hypothesis, in the order of increasing complexity, include :
• Stuart Ross Taylor: a specialist in the Solar System, firmly convinced by the hypothesis. But its truth is not central in its purpose, writing an introduction to the Solar System and its formation. Taylor concludes that the solar system is probably very unusual, being the result of so many accidents and events.
• Stephen Webb: a physicist, presents and rejects the main candidate solutions for the Fermi paradox. The rare earth hypothesis emerges as one of the only remaining solutions at the end of the book.
• Simon Conway Morris: a paleontologist, argues that evolution is a convergence. Morris devotes chapter 5 to the rare earth hypothesis, and quotes Rare Earth with approval. However, while Morris agrees that the Earth may be the only planet in the Milky Way, harboring complex life, he sees the evolution of complex life in intelligent life as fairly likely, contrary to the views of the world. Ernst Mayr, developed in section 3.2 of the following reference.
• John D. Barrow and Frank J. Tipler (1986. 3.2, 8.7, 9), cosmologists vigorously defend the hypothesis that humans are probably the only intelligent beings in the Milky Way, and perhaps the entire universe . But this hypothesis does not constitute the central point of their book, a very attentive study of the anthropic principle and the way in which physical laws have acted particularly favorably to the emergence of complexity in nature.
• Ray Kurzweil, a self-proclaimed pioneer of computer science and singularitarian, argues in Humanity 2.0 that the next singularity demands that the Earth be the first planet on which evolved life has evolved, based on the senses and using technologies. Although other Earth-like planets may exist, the Earth must be the most evolutionarily advanced, otherwise we would have seen obvious signs that another culture has experienced the Singularity and has spread to exploit all the computational capacity of the universe.
Reviews
Criticisms of the rare earth hypothesis take a variety of forms.
Exoplanets are common
More than 300 exoplanets were known in early 2009, and there were more than 800 by the end of 2012. Dr. Alan Boss of the Carnegie Institution of Science estimates that there may be "100 billion" of "terrestrial" planets in our only Galaxie. Dr. Boss thinks that many of them can harbor simple life forms and there could be hundreds of civilizations in our galaxy. Dr. Boss estimates that each star similar to the Sun has an average of a planet similar to the Earth.
"Recent work at the University of Edinburgh has attempted to quantify how many intelligent civilizations exist. The research concluded that there could be thousands. "
Biology of evolution
At the center of the rare earth hypothesis is the requirement for the biology of evolution that, while microbes of all kinds may very well be common in the universe, the existence of complex life is unlikely. However, to date, the only evolutionary biologist who has commented on this point is Simon Conway Morris in 2003. The hypothesis concludes, more or less, that complex life is rare because it can only evolve 'on the surface of a planet similar to the Earth or a suitable satellite of a planet. Some biologists, such as Jack Cohen, think this statement too restrictive and unimaginative: he sees it as a form of circular reasoning (see Hypothetical biochemistry, a speculative biochemistry on alien lifeforms). Earth-like planets may indeed be very rare, but the emergence of a complex life not based on carbon chemistry could occur in other environments.
David Darling
According to Darling, the rare earth hypothesis is neither a hypothesis nor a prediction, but hardly a description of how life appeared on Earth. In his view, Ward and Brownlee did nothing more than select the factors that best suited their hypothesis:
"What matters is not whether there is anything unusual about the Earth: there will be idiosyncratic aspects for each of the planets in space. What matters is whether one of the circumstances is not only unusual but also essential for the complex life. So far, we have not seen anything that suggests it. "
Richard Dawkins
There are actually many planets and some will inevitably be similar to Earth :
"... The anthropogenic alternative to the hypothesis of design is statistical. Scientists evoke the magic of big numbers. It has been estimated that there are between 100 and 300 billion stars in the galaxy and around 100 billion galaxies in the universe. By eliminating a few zeros under ordinary caution, a billion billion remains a reasonable estimate of the number of planets in the universe. Suppose now that the origin of life, the spontaneous appearance of something equivalent to DNA, was really an event of stupendous improbability. Suppose it was so unlikely that it could only happen once on a billion planet, (...). But here we are talking about the probability of one in a billion. And yet, even with such absurdly reduced probabilities, life would still have appeared on a billion planets, among which, of course, is the Earth.
The conclusion is so surprising that I will repeat it. If the probability of spontaneous occurrence of life on a planet was one to one billion, despite this, this unlikely event to a staggering degree would still occur on a billion planets. The probability of finding any of this billion living planets reminiscent of the proverbial needle with its haystack, But we do not need to change our habits to find a needle, since (back to the anthropic principle) any being able to engage this research must necessarily be seated on one of these prodigiously rare needles even before starting it. "
— Richard Dawkins, The God Delusion
Physics and cosmology
If the following suggestions are correct, other parts of the universe or multiverse are likely to harbor life.
Quantum mechanics for many worlds
The theory of multiple worlds produces at least one planet similar to the Earth in certain chronologies, or certain worlds. In this context, a timeline means a world or parallel universe. This applies even in a universe where the probability of forming only one is small. The Big Bang chronology is believed to have begun about 13.7 billion years ago, The Solar System was formed nearly 4.6 billion years ago; during the 9 billion years between the formation of the Universe and the formation of the Solar System, an uncountable quantity of copies of the universe have developed. One can reasonably expect the development of at least one solar system among all these copies of the universe. Similarly, since the Earth and the solar system have been growing for 4.6 billion years, copies of the Earth have increased exponentially, so it is not surprising that at least one of them has grown into view of an intelligent life.
If the rare earth hypothesis and the multiple world theory are both exact, then the presence of intelligent observers is assured, even if the rare earth equation is close to zero. But such lands will almost always be isolated, which responds to the Fermi Paradox. The rare earth hypothesis may not be true, as discussed above.
The theory of multiple worlds alone can not so easily explain the seemingly finely tuned Universe. In most versions of multiple worlds, the physical constants of all worlds are similar.
F I N.
Hypothesis of the rare earth.

Are the planets able to shelter life like the Earth rare?
In planetary astronomy and astrobiology, the rare earth hypothesis holds that the emergence of a complex multicellular life (metazoa) on Earth has required an unlikely combination of astrophysical and geological events and circumstances. The term rare earth comes from Rare Earth: Why Complex Life Is Uncommon in the Universe (2000), a book written by geologist and paleontologist Peter Ward, and astronomer and astrobiologist Donald E. Brownlee. Their book is the source of the essence of this article.
The rare earth hypothesis is opposed to the principle of mediocrity (also called Copernican principle) supported by Carl Sagan and Frank Drake, among others1. The principle of mediocrity concludes that the Earth is a typical rocky planet, in a typical planetary system, located in a region without any exceptional character of a current barred spiral galaxy. It is therefore likely that the universe teems with complex life. Ward and Brownlee argue that planets, planetary systems, and galactic regions that support a complex life, such as the Earth, the Solar System, and our Milky Way region, are probably very rare.
The rare earth hypothesis, concluding that complex life is not common, is a possible solution to the Fermi paradox: "If extraterrestrials are common, why do not we see them? "
Galactic habitable areas
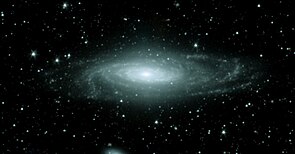
In a zone lacking metals or near the center of the galaxy and exposed to significant radiation, a planet is not able to shelter life. The galaxy photographed is NGC 7331, often identified as the twin of the Milky Way.
Rare Earth suggests that much of the known universe, including large parts of our galaxy, can not harbor complex life; Ward and Brownlee refer to these areas as "dead zones". Parts of galaxies where complex life is possible constitute the habitable galactic zone. This area is primarily a function of the distance to the galactic center. With increasing distance:
1. The metallicity of stars declines, and metals (term which, in astronomy, means elements heavier than hydrogen and helium) are necessary for the formation of telluric planets.
2. X and gamma radiation from black holes in the center of the galaxy, and near neutron stars, are losing intensity. A radiation of this nature is considered dangerous for the complex life, so that the rare earth hypothesis predicts that the primitive universe, and also now, the regions of the galaxy where the stellar density is high and the supernovae are unsuited to the development of complex life.
3. The probability of gravitational disturbances to planets (and planetesimals) by nearby stars decreases with the decreasing density of stars. So the more distant a planet is from the center of the galaxy, the less likely it is to hit a big car. A sufficiently large impact can lead to the extinction of all complex life on a planet.
Proposition (1) above governs the outer reaches of a galaxy; propositions (2) and (3) govern the inner regions of the galaxy, the globular clusters and the spiral arms of the spiral galaxies. These arms are not physical objects, but regions of a galaxy characterized by a high rate of star formation, moving at a speed of about 240 km / s (for the solar system), they describe a movement wave. From the center of a galaxy to its periphery, the capacity to shelter life increases and then falls. So that the habitable galactic zone can affect the shape of a ring, sandwiched between its center and its periphery, both uninhabitable.
Not only can a planetary system enjoy a location favorable to the appearance of complex life, but it must also maintain this location for a period long enough for the complex life to evolve. Thus, a central star with a galactic orbit that evolves away from galactic regions with high levels of radiation such as galactic centers and spiral arms seems potentially a very favorable support. If the galactic orbit of the central star is eccentric, elliptical or hyperbolic, it will cross a few spiral arms, but if the orbit is a nearly perfect circle, and the orbital velocity equals the speed of "rotation" of the spiral arms, the star will eventually sink into a spiral arm region, first gradually, then fully. Therefore, the supporters of the "Rare Earth" conclude that a life-supporting star must have a nearly circular galactic orbit around the center of its galaxy. The required synchronization between the orbital velocity of a central zone star and the velocity of the spiral arms wave can occur only in rather narrow bands of distances to the galactic center. These regions are referred to as the "habitable galactic zone". Lineweaver et al. calculate that the habitable galactic zone is a ring of 7 to 9 kiloparsec in diameter, which comprises not more than 10% of the stars of the Milky Way. Based on conservative estimates of the total number of stars in the galaxy, this could be in the range of 20 to 40 billion stars. Gonzalez et al. reduce this figure by half, they estimate that at most 5% of the stars of the Galaxy are in the galactic zone habitable.
Indeed, the Sun's orbit around the center of the Milky Way is a nearly perfect circle, with a period of revolution of 225 million years, closely matching the rotation period of the galaxy. Karen Masters has calculated that the Sun's orbit crosses a major spiral arm every 100 million years. On the contrary, the rare earth hypothesis predicts that the sun, since its formation, should not have passed through any spiral arms. However, some researchers have suggested that several mass extinctions actually correspond to previous spiral arm crossings.
A central star with the adequate character
The example of the Earth suggests that complex life requires the presence of water in the liquid state and its planet must therefore be at the appropriate distance from its star. This is the heart of the concept of habitable zone or principle of Goldilocks (in). The habitable zone forms a ring around the central star. If the orbit of a planet is too close to or too far from its sun, the temperature of its surface is incompatible with water in liquid form (although water below the surface, as has been suggested for satellites Europe and Enceladus, as well as for the dwarf planet Ceres can exist in different places). Kasting et al. (1993) estimate that the habitable zone of the Sun extends between 0.95 and 1.15 astronomical units.
The living area varies with the type and age of the central star. The habitable zone of a star in the main series drifts with time until the star becomes a white dwarf, when the habitable zone disappears. The habitable zone is closely linked to the greenhouse effect caused by atmospheric carbon dioxide (CO2) or other greenhouse gases, mainly water vapor in the case of the Earth. The collective effect of these gases increases the average temperature on Earth by approximately 33 ° C compared to what it would otherwise be.
It is assumed that a star must have rocky planets within its habitable zone. While the habitable zone of hot stars such as Alpha Canis Majoris (Sirius) or Alpha Lyrae (Vega) is wide, there are two problems :
1. Since it was thought (at the time of writing "Rare Earth") that the rock planets were forming closer to their central stars, the candidate planets would then form too close to the star to find themselves in the habitable zone. This does not solve life on a gas giant moon. Hot stars also emit a lot more ultraviolet radiation that will ionize any planetary atmosphere.
2. The hot stars mentioned above have a short life span, becoming red giants in less than a billion years. This time is not enough for an advanced life to evolve. These considerations remove stars of types F6 to O (see spectral type as the focus of evolution of an evolved metazoan life.
On the other hand, small stars (red dwarfs) have small radius living areas. This proximity means that one side of the planet is constantly facing the star, and the other remains permanently in the dark, a phenomenon of "lock" due to the effects of tidal forces. This "tidal locking" of a hemisphere from a planet to its primary body will make the diurnal face of the planet constantly hot, while the night face will remain extremely cold, even cold enough so that most atmospheric gases can freeze! Planets within a liveable area with a small radius are also subject to increased risks from solar flares (see Aurelia), which will tend to ionize the atmosphere and be unfavorable to the complex life of the planet. multiple ways. Proponents of "Rare Earth" hold that this eliminates the possibility of living in such systems, while exobiologists have suggested that livability may exist in the correct circumstances. This is a central blocking point for the theory, since these late K-type stars and M constitute about 82% of all hydrogen-consuming stars.
Proponents of "Rare Earth" argue that the type of central stars that are "just right" ranges from F7 to K1. Such stars are not common: G-type stars like the Sun (between the warmer F and the colder K) only comprise 9% of the hydrogen-consuming stars in the Milky Way.

Globular clusters probably do not house life.
Older stars such as red giants and white dwarfs probably do not contain life either. Red giants are common in globular clusters and elliptical galaxies. White dwarfs are essentially dying stars that have already gone through their red giant phase. The diameter of a red giant has increased substantially since his youth. If a planet has been in the habitable zone of a star during its youth and middle age, it will be burnt when the star becomes a red giant (although theoretically the planets farther away might have become habitable) .
The energy expenditure of a star throughout its life changes only very gradually: variable stars such as, for example, the Cepheid variables are extremely unlikely hosts of life. If the energy expenditure of the central star decreases suddenly, even for a relatively short time, the planet's water can freeze. On the other hand, if the energy expenditure of the central star increases significantly, the oceans can evaporate, which produces a greenhouse effect: this phenomenon can eliminate any reformation of the oceans.
There is no known way to achieve life without complex chemistry, and such chemistry requires metals (which means heavier elements than hydrogen, helium and lithium). This suggests that one of the conditions for life is a solar system rich in metals. The only known mechanism for the creation and dispersion of metals is a supernova explosion. The presence of metals in the stars is revealed by their absorption spectrum, and stellar spectra studies reveal that many stars, and perhaps most of them, are low in metals. Low metallicity characterizes the primordial universe, globular clusters and other stars formed during the youth of the universe, stars in most other galaxies as large spiral galaxies, and stars in the outer regions of all galaxies . Thus, metal-rich central stars capable of harboring a complex life are estimated to be very common in the quiet suburbs of larger spiral galaxies, hospital regions with complex life, for another reason, specifically the absence of high radiation.
A planetary system
A cloud of gas capable of giving birth to a star can also give birth to a gaseous planet with low metallicity (Jovian) like Jupiter and Saturn. But the Jovian planets do not have a firm surface, considered necessary for the complex life (their satellites, however, can have hard surfaces). According to Ward and Brownlee's argument, a planetary system capable of harboring complex life must be structured more or less like the Solar System, with the small rock planets inside and the Jovian planets outside. However, recent research questions this line of argument.
Uncertainty about Jupiter

Jupiter is the fifth planet from the Sun and the largest planet inside the Solar System.
At the time of Ward and Brownlee's book, gaseous giants were seen as helping to sustain life, retaining distant asteroids from the planets that harbor life. So it was thought that a gas giant was protecting inner rocky planets against asteroid bombardment. However, recent computer simulations on the subject suggest that the situation is more complex: it appears that Jupiter causes three times more impacts than it avoids, while the replacement of Jupiter by a body the size of Saturn , would double the rate of bombing compared to that obtained with Jupiter.
Orbital disturbance
A gas giant must not be too close to a body on which life develops, unless that body is one of its moons. The position too close to a gas giant could disrupt the orbit of a potentially life-giving planet, either directly or by drifting into the habitable zone.
Newtonian dynamics can produce chaotic planetary orbits, especially in a system with large planets with high orbital eccentricity.
The need for a stable orbit is imposed on extra-solar planetary systems composed of large planets with orbits near the star, known as hot Jupiters. Hot Jupiter are thought to have formed much larger distances from their parent-star than they currently are, and have migrated inward to their present position. In this process, they will have catastrophically disrupted the orbit of all planets in the habitable zone.
Global dimension
A planet too small is not able to retain enough atmosphere. Changes in surface temperature would be much larger, and the average temperature would decrease. The formation of substantial oceans and their long-term maintenance would be compromised. The surface of a small planet will also tend to be very rugged, with high mountains and deep canyons. His heart will cool too quickly, and plate tectonics either will not last as long as on a larger planet, or will not occur at all.
According to astronomer Michaël Meyer of the University of Arizona, small planets like the Earth may be common: "Our observations suggest that between 20% and 60% of Sun-like stars show signs of rocky planets, little different from the processes we think they led to the appearance of planet Earth. It's very exciting. ". The team led by Meyer has highlighted the presence of cosmic dust near solar-type stars, and sees this as a by-product of rocky planet formation.
A big moon
The Moon is unusual because the other rocky planets of the Solar System either do not have a natural satellite (Mercury and Venus), or have tiny, presumably captured asteroids (Mars).
The theory of giant impact formulates the hypothesis that the Moon results from the impact of the planet Theia (the size of Mars) with the Earth during its youth. This giant collision also gave the Earth its inclination of the axis and its speed of rotation. A fast rotation reduces the temperature change over the course of a day and makes photosynthesis viable. The rare earth hypothesis continues with the argument that the inclination of the axis should not be too great in relation to the orbital plane. A planet with too much inclination would withstand extreme seasonal climatic variations, unfavorable to complex life. A planet with little or no inclination would lack a stimulus to evolution provided by climatic variations. In this perspective, the tilt of the Earth is "just right". The gravity of big satellites also stabilizes the tilt of the planet; without this influence, the inclination would become chaotic, possibly rendering impossible the complex terrestrial forms of life.
If the Earth did not have a moon, the ocean tides resulting solely from the gravitational influence of the Sun would be very modest. A large satellite engenders the existence of residual pools, which may be essential to the formation of complex life.
A large satellite also increases the likelihood of plate tectonics by the effect of tidal forces on the planet's crust. The impact of the Moon may also have had an initiating role of plate tectonics, without which the continental crust would cover the entire planet, leaving no room for the oceanic crust [ref. necessary]. It is possible that large-scale convection of the mantle, necessary prior to the appearance of plate tectonics, could not have emerged in the absence of inhomogeneity of the crust. However, there are strong signs that plate tectonics existed on Mars in the past, without such a mechanism initiating it.
If a giant impact is the only way for an inner rocky planet to acquire a big satellite, any planet in the circumstellar habitable zone will need to form as a double planet, so that there is a sufficiently massive object to generate an impact to generate a large satellite in a timely manner. An "impacting" object of this nature is not necessarily improbable. Recent work by Edward Belbruno and Princeton University's John Richard Gott suggests that an adequate "impacting" body could be formed at Lagrange points on a planet.
Plate tectonics
A planet can only have tectonic plates if its chemical composition allows it. The only known long-term source providing the necessary heat is the radioactivity that occurs in the depths of the planet. The continents must also consist of granitic rocks that float on denser basaltic rocks. Taylor points out that subduction zones (an essential part of tectonic plates) require the lubricating action of large amounts of water; on Earth such areas exist only in the ocean floor.
Episode of the inertial upheaval
There is enough evidence that the rate of continental drift during the Cambrian explosion was abnormally high. In fact, the continents moved from the Arctic to the equator and vice versa in 15 million years or less. Kirschvink et al. have proposed the following controversial explanation: a 90 ° change in the axis of rotation of the Earth, caused by an imbalance of the continental masses with respect to the axis. The result was an enormous change in climate, ocean currents, and so on, occurring in a very short time and affecting the entire Earth. They named their explanation as "the episode of inertial upheaval".
This scenario has not yet been endorsed by the scientific community, but if such an event really happened, then, it was very implausible, and if it was a necessity for the evolution of an animal life more complex than sea sponges or coral reefs, then we still get a new reason for life (complex?) to be rare in the universe.
Rare Earth equation
The following is adapted from Cramer. The rare earth equation is the riposte
from Ward and Brownlee to Drake's. It allows the calculation of N, the number of Earth-like planets in the Milky Way with complex life forms.
The rare Earth equation, unlike Drake's, does not factor in the probability that complex life evolves into intelligent life that discovers technology (remember that Ward and Brownlee are not evolutionary biologists). Barrow and Tipler observe the consensus among these biologists that the path of evolution from primitive Cambrian chordates (eg Pikaia) to homo sapiens was a highly improbable event. For example, the large brains of humans are the mark of adaptive disadvantage, since they require an expensive metabolism, a long period of gestation, and a duration of youth of more than 25% of the average total duration of the life. Other improbable traits in humans include :
• Being the only vertebrate non-avian terrestrial bipedarian of great extent. Combined with an unusual eye-hand coordination, this allows skillful manipulation of the physical environment with the hands;
• A vocal apparatus much more expressive than that of any other mammal, allowing speech. Speech allows humans to interact cooperatively, share knowledge, and acquire a culture;
• The ability to formulate abstractions to a degree that allows the invention of mathematics, and the discovery of science and technology. It must be kept in mind that humans have recently acquired all that makes up the sophistication of their scientific and technological knowledge.
Supporters
Books supporting the rare earth hypothesis, in the order of increasing complexity, include :
• Stuart Ross Taylor: a specialist in the Solar System, firmly convinced by the hypothesis. But its truth is not central in its purpose, writing an introduction to the Solar System and its formation. Taylor concludes that the solar system is probably very unusual, being the result of so many accidents and events.
• Stephen Webb: a physicist, presents and rejects the main candidate solutions for the Fermi paradox. The rare earth hypothesis emerges as one of the only remaining solutions at the end of the book.
• Simon Conway Morris: a paleontologist, argues that evolution is a convergence. Morris devotes chapter 5 to the rare earth hypothesis, and quotes Rare Earth with approval. However, while Morris agrees that the Earth may be the only planet in the Milky Way, harboring complex life, he sees the evolution of complex life in intelligent life as fairly likely, contrary to the views of the world. Ernst Mayr, developed in section 3.2 of the following reference.
• John D. Barrow and Frank J. Tipler (1986. 3.2, 8.7, 9), cosmologists vigorously defend the hypothesis that humans are probably the only intelligent beings in the Milky Way, and perhaps the entire universe . But this hypothesis does not constitute the central point of their book, a very attentive study of the anthropic principle and the way in which physical laws have acted particularly favorably to the emergence of complexity in nature.
• Ray Kurzweil, a self-proclaimed pioneer of computer science and singularitarian, argues in Humanity 2.0 that the next singularity demands that the Earth be the first planet on which evolved life has evolved, based on the senses and using technologies. Although other Earth-like planets may exist, the Earth must be the most evolutionarily advanced, otherwise we would have seen obvious signs that another culture has experienced the Singularity and has spread to exploit all the computational capacity of the universe.
Reviews
Criticisms of the rare earth hypothesis take a variety of forms.
Exoplanets are common
More than 300 exoplanets were known in early 2009, and there were more than 800 by the end of 2012. Dr. Alan Boss of the Carnegie Institution of Science estimates that there may be "100 billion" of "terrestrial" planets in our only Galaxie. Dr. Boss thinks that many of them can harbor simple life forms and there could be hundreds of civilizations in our galaxy. Dr. Boss estimates that each star similar to the Sun has an average of a planet similar to the Earth.
"Recent work at the University of Edinburgh has attempted to quantify how many intelligent civilizations exist. The research concluded that there could be thousands. "
Biology of evolution
At the center of the rare earth hypothesis is the requirement for the biology of evolution that, while microbes of all kinds may very well be common in the universe, the existence of complex life is unlikely. However, to date, the only evolutionary biologist who has commented on this point is Simon Conway Morris in 2003. The hypothesis concludes, more or less, that complex life is rare because it can only evolve 'on the surface of a planet similar to the Earth or a suitable satellite of a planet. Some biologists, such as Jack Cohen, think this statement too restrictive and unimaginative: he sees it as a form of circular reasoning (see Hypothetical biochemistry, a speculative biochemistry on alien lifeforms). Earth-like planets may indeed be very rare, but the emergence of a complex life not based on carbon chemistry could occur in other environments.
David Darling
According to Darling, the rare earth hypothesis is neither a hypothesis nor a prediction, but hardly a description of how life appeared on Earth. In his view, Ward and Brownlee did nothing more than select the factors that best suited their hypothesis:
"What matters is not whether there is anything unusual about the Earth: there will be idiosyncratic aspects for each of the planets in space. What matters is whether one of the circumstances is not only unusual but also essential for the complex life. So far, we have not seen anything that suggests it. "
Richard Dawkins
There are actually many planets and some will inevitably be similar to Earth :
"... The anthropogenic alternative to the hypothesis of design is statistical. Scientists evoke the magic of big numbers. It has been estimated that there are between 100 and 300 billion stars in the galaxy and around 100 billion galaxies in the universe. By eliminating a few zeros under ordinary caution, a billion billion remains a reasonable estimate of the number of planets in the universe. Suppose now that the origin of life, the spontaneous appearance of something equivalent to DNA, was really an event of stupendous improbability. Suppose it was so unlikely that it could only happen once on a billion planet, (...). But here we are talking about the probability of one in a billion. And yet, even with such absurdly reduced probabilities, life would still have appeared on a billion planets, among which, of course, is the Earth.
The conclusion is so surprising that I will repeat it. If the probability of spontaneous occurrence of life on a planet was one to one billion, despite this, this unlikely event to a staggering degree would still occur on a billion planets. The probability of finding any of this billion living planets reminiscent of the proverbial needle with its haystack, But we do not need to change our habits to find a needle, since (back to the anthropic principle) any being able to engage this research must necessarily be seated on one of these prodigiously rare needles even before starting it. "
— Richard Dawkins, The God Delusion
Physics and cosmology
If the following suggestions are correct, other parts of the universe or multiverse are likely to harbor life.
Quantum mechanics for many worlds
The theory of multiple worlds produces at least one planet similar to the Earth in certain chronologies, or certain worlds. In this context, a timeline means a world or parallel universe. This applies even in a universe where the probability of forming only one is small. The Big Bang chronology is believed to have begun about 13.7 billion years ago, The Solar System was formed nearly 4.6 billion years ago; during the 9 billion years between the formation of the Universe and the formation of the Solar System, an uncountable quantity of copies of the universe have developed. One can reasonably expect the development of at least one solar system among all these copies of the universe. Similarly, since the Earth and the solar system have been growing for 4.6 billion years, copies of the Earth have increased exponentially, so it is not surprising that at least one of them has grown into view of an intelligent life.
If the rare earth hypothesis and the multiple world theory are both exact, then the presence of intelligent observers is assured, even if the rare earth equation is close to zero. But such lands will almost always be isolated, which responds to the Fermi Paradox. The rare earth hypothesis may not be true, as discussed above.
The theory of multiple worlds alone can not so easily explain the seemingly finely tuned Universe. In most versions of multiple worlds, the physical constants of all worlds are similar.
F I N.